What are Laser Gain Crystals?
Laser Gain crystals are the components within solid-state lasers which enable the amplification of light through stimulated emission – the process which forms the basis of laser operation. These gain media consist of a host crystal or glass matrix doped with rare earth or transition metal ions. The exact combination of crystal and ion determines the specific laser output properties it can support.
Laser gain crystals are the heart of solid-state lasers, providing the medium in which the generation and amplification of light occurs. Gain crystals consist of two key components. The first is a host material – usually a crystal or sometimes glass. The second is a dopant ion, invariably of a rare-earth or transition metal element.
Gain crystals must perform at least two of the basic functions required for laser operation. First, they must absorb pump energy. Second, they must be capable of maintaining a population inversion in order to support stimulated emission. In some cases, the gain crystal may also function as part of the resonant cavity.
Because all solid-state gain crystals are electrical insulators, they can only be optically pumped. The dopants absorb this pump light energy which excites them to higher energy levels. When these excited ions return to their ground state, they emit photons in a process known as stimulated emission. This process is amplified within the laser cavity, resulting in the generation of coherent laser light. The specific characteristics of the laser, including its wavelength and energy conversion efficiency, depend on the choice of the dopant and the host crystal.
Crystal Characteristics
There are several factors that influence the choice of host crystal for a specific laser type or application. These include the material’s optical transparency, thermal conductivity, mechanical strength, and chemical stability, all of which are crucial for efficient laser operation.
An ideal host crystal should possess a wide transparency range to allow for efficient transmission of the laser wavelength, minimizing intrinsic absorption that could lead to unwanted heating. High thermal conductivity is another vital property, as it enables the host crystal to dissipate heat generated during the laser pumping and operation processes effectively, maintaining stable laser performance and preventing thermal lensing or damage.
Moreover, mechanical strength and chemical stability are essential for ensuring the longevity and durability of the laser system, especially in demanding environmental conditions or high-power applications. The host crystal should be resilient to thermal shock and resistant to degradation or damage from external chemical agents.
Additionally, the crystal lattice of the host material must be compatible with the dopant ions, allowing for their uniform distribution within the crystal structure without causing significant lattice distortion. This compatibility is critical for achieving efficient dopant excitation and energy transfer processes, which are fundamental for stimulated emission and laser action. The chart summarizes the compatibility of the most common laser crystals and dopants.
Host Material |
Dopant |
|||||
Rare Earth Elements |
Transition Metals |
|||||
|
Nd |
Yb |
Er |
Tm |
Cr |
Ti |
YAG (Y₃Al₅O₁₂) |
✓ |
✓ |
✓ |
✓ |
✓ |
|
YVO₄ |
✓ |
✓ |
|
|
|
|
Glass |
✓ |
✓ |
✓ |
✓ |
|
|
YLF (LiYF₄) |
✓ |
✓ |
✓ |
✓ |
|
|
Sapphire (Al₂O₃) |
|
|
|
|
✓ |
✓ |
Chalcogenide |
|
|
✓ |
✓ |
|
|
Fluoride |
|
✓ |
✓ |
✓ |
|
|
Commonly Used Crystals
There is quite a variety of laser crystals in current use, as well as others that have come and gone in popularity over the years. But there are a few that that dominate the market, and service the bulk of solid-state laser applications.
The Yttrium Aluminum Garnet (YAG) family includes some of the most widely used industrial and medical laser gain crystals (especially Nd:YAG). YAG supports a variety of dopants like Neodymium (Nd), Ytterbium (Yb), Erbium (Er), Thulium (Tm), and Chromium (Cr).
These dopants endow the YAG crystal with specific properties, including high efficiency. YAG also offers excellent thermal conductivity, mechanical durability, and a broad transparency range. Plus, YAG can produce high-peak-power pulses when used with passive Q-switches. Together, these properties make YAG an ideal host material for many medical, industrial, and scientific applications.
The Vanadate family, particularly Nd:YVO₄, stands out for its high gain and excellent pump light absorption properties, making it highly efficient, especially in diode-pumped laser systems. This efficiency also ensures that, even at lower power levels, the laser can produce a high-quality beam that is capable of precise and clean cuts or marks. The high absorption allows for shorter crystal lengths and more compact laser designs.
However, compared to other laser gain crystals like those in the YAG family, Vanadate crystals have lower thermal conductivity. This can limit their performance in high-power applications due to increased susceptibility to thermal effects like lensing and birefringence. This characteristic necessitates careful thermal management to maintain optimal laser performance.
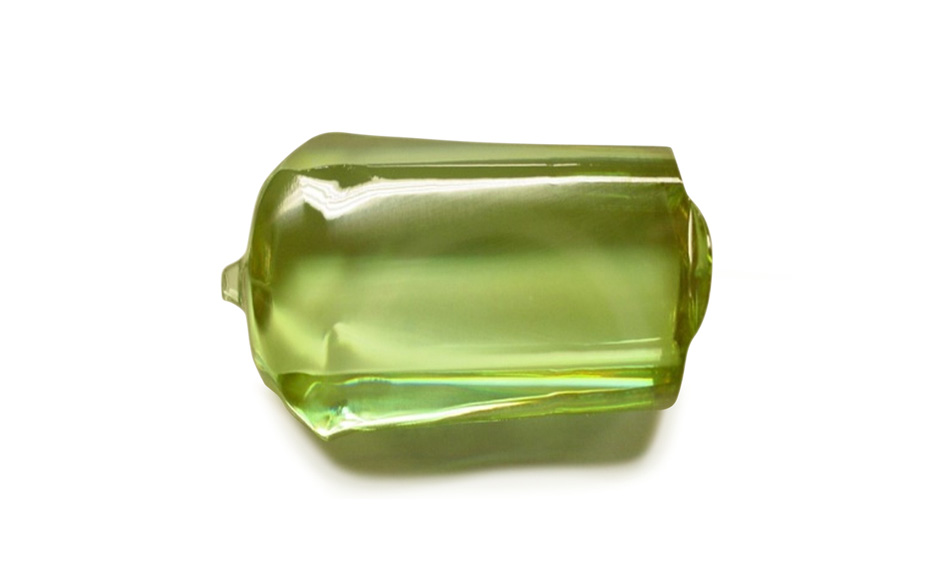
Nd:YVO₄ is grown in boules from which individual laser components are cut and polished.
As a result, the Vanadate family remains a popular choice for applications requiring high beam quality and efficiency in a compact form factor. However, they may not be the first choice for high-power or high-energy applications where thermal management becomes more critical.
Sapphire, specifically Ti:Sapphire, stands out in laser technology for its broad tunability, spanning approximately 650 nm to 1100 nm. This broad gain bandwidth also makes Ti:Sapphire lasers capable of producing extremely short pulses, down to the femtosecond range. Because of these characteristics, Ti:Sapphire is the first choice for the most demanding, high-performance, ultrafast lasers and amplifiers, such as the Coherent Vitara and Astrella.
Despite these advantages, Ti:Sapphire lasers have some limitations. In particular, they need high-power pump sources, such as solid-state green lasers, for efficient operation. This requirement can increase laser system cost and complexity.
Glass has a disordered, amorphous arrangement of atoms. In contrast, crystals have a highly ordered, repeating atomic structure that extends throughout the material. As a result, glass, particularly when doped with rare-earth elements like Nd, Er, or Yb, offers a unique set of properties as a laser gain medium.
One of the key advantages of glass hosts is their broad emission spectra, which support wide tunability and the generation of ultra-short laser pulses. This characteristic is especially beneficial for applications requiring flexible wavelength output or short pulse durations, such as in medical devices, telecommunications, and fundamental research. Furthermore, glass materials can be produced in large sizes and various shapes, providing versatility in laser design. For example, very large Nd:glass slabs are used in high-energy laser systems, such as those for laser fusion experiments.
However, glass hosts have lower thermal conductivity compared to crystalline materials like YAG. This can limit their power scaling capabilities due to increased susceptibility to thermal effects. This lower thermal performance necessitates careful management of heat generation and removal in high-power applications. Additionally, the lower gain per unit length of glass, compared to crystalline hosts, often requires longer gain media, which can increase the complexity and size of the laser system.
Dopant Selection
Rare earth and transition metal ions are the most commonly used dopants in laser gain media due to their unique electronic structures which provide several advantageous optical properties for laser operation.
Rare earth ions have well-defined, sharp energy levels because their valence electrons are in 4f atomic orbitals, which are shielded by the outer 5s and 5p electrons. This shielding minimizes interaction with the host lattice, leading to minimal broadening of energy levels and enabling precise control over the laser emission wavelength. This also translates to fewer non-radiative decay processes, resulting in higher quantum efficiency (conversion of absorbed pump energy into laser light). The electronic transitions of these ions are less affected by changes in the host material or temperature, making lasers based on these dopants stable and reliable under various conditions.
Conversely, transition metal ions have their valence electrons in the 3d orbital, which is less shielded by their outer 4s electron shells. This means their energy levels are more strongly influenced by the host material, leading to broader absorption and emission bands. These broader bands can be advantageous because they make transition metal ions compatible with a variety of laser pumping schemes, allowing for greater versatility in laser design. They can also provide broader gain bandwidth, which allows for tunable laser operation over a wider range of wavelengths.
Rare earth ions, especially Er and Tm, tend to emit in the near-infrared through mid-infrared regions. Transition metal ions can offer laser operation in the visible to near-infrared spectrum. Ti is notable for its exceptionally wide tunability across the visible to near-infrared range.
The rare earth ion Yb stands out amongst all others for several reasons, which is why there are so many popular laser gain crystals in the Yb-doped family. For one, Yb ions have a relatively simple energy level structure. Specifically, the Yb³⁺ ion has just a single electron in the 4f shell. This results in efficient absorption and emission processes. This simplicity allows for high power efficiency with minimal loss.
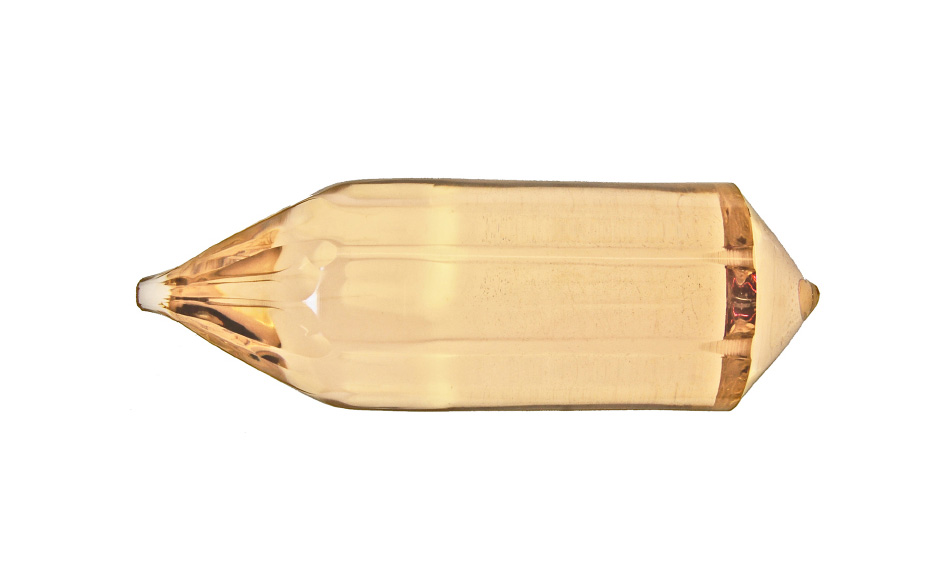
Boule of Yb-doped material before cutting and polishing.
Additionally, Yb-doped materials exhibit a wide absorption bandwidth, allowing for greater flexibility in the choice of pump sources and enabling the generation of ultra-short pulses. For example, Yb-doped crystals can be effectively pumped with readily available and inexpensive diode lasers at wavelengths around 980 nm. This further enhances their efficiency and reduces operational costs.
Growing Laser Gain Crystals
The production of laser gain crystals involves sophisticated growth and doping techniques to ensure the precise distribution of dopant ions within the host crystal and to achieve the desired optical and physical properties. Although all laser gain crystal manufacturers use fundamentally similar production methods, significant differences exist in their proprietary knowledge, quality control procedures, process control instrumentation, and metrology tools. These ultimately lead to significant variations amongst manufacturers in quality, underlining the fact that not all laser gain crystals are created equal.
One common crystal growth method is the Czochralski process. This involves melting the host material along with the dopant in a crucible and then slowly pulling a seed crystal from the melt, allowing a new crystal to grow on it. This method allows for careful control over the crystal's composition and structure. Nd:YAG and Er:YAG are two crystals often produced using the Czochralski method.
The Bridgman-Stockbarger method is another widely used crystal growth technique. It is particularly effective for producing single-crystal materials with minimal defects. A key reason for this is that the Bridgman-Stockbarger technique minimizes the thermal gradient (temperature difference between the molten zone and the solidification front) during crystal growth.
The Bridgman-Stockbarger process begins by placing the raw materials – host material and dopants – inside a sealed crucible. This crucible is then slowly lowered through a furnace with a carefully controlled temperature gradient – typically a hotter region at the top and a cooler region at the bottom.
As the crucible moves from the hotter to the cooler region, the material inside starts to melt in the upper (hotter) zone of the furnace. As it is further lowered into the cooler zone, the molten material begins to solidify from the bottom, or around a seed crystal placed at the bottom of the melt. This directional solidification helps in forming a single crystal, as the crystal grows along the thermal gradient from the cooler end upwards. The Bridgman-Stockbarger technique is usually employed for growing crystal materials that have high melting points, when the crystal growth requires a specific orientation, or for larger boules that are difficult to achieve with the Czochralski process.
Learn more about Coherent crystals.