Laser Cooling
What is Laser Cooling?
Laser cooling is a technique in atomic physics and quantum optics that can slow down and trap atomic and molecular particles. The method is based on the interaction between light and matter, and it exploits the way in which photons transfer momentum to atoms.
The basic principle of laser cooling is the absorption and re-emission of photons. When an atom absorbs a photon, its energy is increased, and it moves to a higher energy level. When it later re-emits the photon, it loses energy and falls back to a lower energy level. The key to laser cooling is to ensure that the atom always re-emits the photon in a direction that is opposite to its motion. This means that, on average, the atom loses more momentum to the photons than it gains, and it slows down as a result. This allows atoms to be captured in optical traps.
Several Different Methods
There are several different methods of laser cooling, each of which is suited to different types of particles. The most common method is Doppler cooling, which is used for cooling neutral atoms. Doppler cooling relies on the fact that the frequency of the light absorbed by an atom depends on its velocity. As an atom moves towards a laser, the frequency of the light it absorbs is shifted to a higher value, and as it moves away from the laser, the frequency is shifted to a lower value. By using two or more lasers that are detuned from each other, it is possible to ensure that the atom always re-emits photons in a direction that opposes its motion, leading to cooling.
Another method of laser cooling is known as Sisyphus cooling. This technique is used for cooling ions, which are charged particles. Sisyphus cooling relies on the interaction between the electric field of the light and the charge of the ion. When an ion moves through a laser beam, it experiences a time-varying force that is proportional to the gradient of the laser intensity. By using two or more lasers that are detuned from each other, it is possible to ensure that the ion always moves uphill in the laser intensity, leading to cooling.
Polarization gradient cooling also uses two counterpropagating laser beams. Here the two beams have orthogonal or opposite polarization states. Some setups use circularly polarized beams, some use linearly polarized beams. In both cases, the cooling mechanism is somewhat complex based on the magnetic properties (Zeeman effect) of closely spaced electron energy states in the atoms. Bottom line – this method can cool atoms to even lower effective temperatures than the more common Doppler cooling method. But the forces involved are very weak, so the atoms have to be pre-cooled or the polarization gradient cannot trap them at all.
In addition to Doppler cooling, Sisyphus cooling, and polarization gradient cooling, there are several other methods of laser cooling, including sub-Doppler cooling and resolved sideband cooling. Each method has its own advantages and disadvantages, and the choice depends on the specific requirements of the experiment.
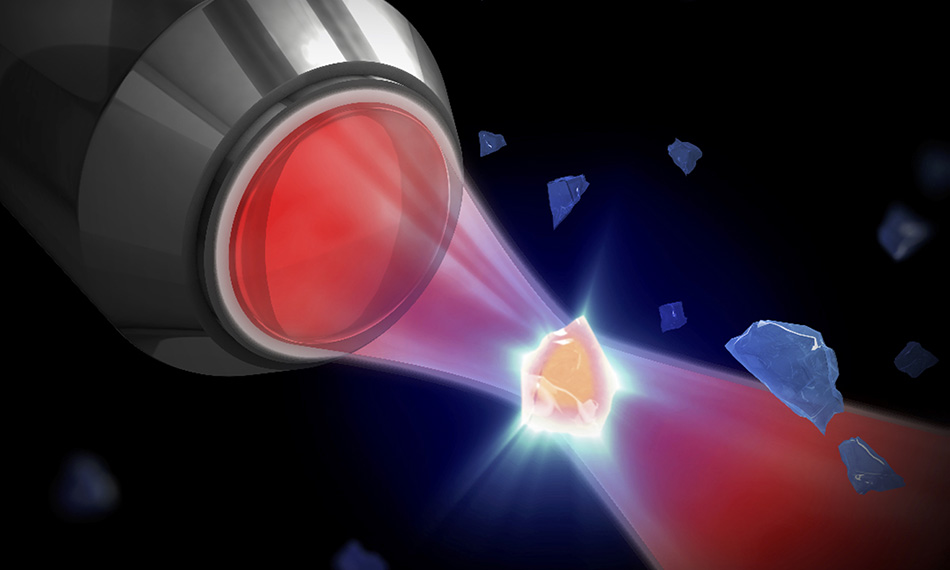
Some Applications of Laser Cooling
The applications of laser cooling are numerous and varied. One of the most important applications is the creation of ultra-cold ensembles of atoms or ions, which are used in many areas of physics and chemistry. For example, ultra-cold atoms can be used to study fundamental quantum phenomena, such as Bose-Einstein condensation and superfluidity. They can also be used to simulate many-body quantum systems, such as materials and magnetic systems. In addition, ultra-cold atoms are used in precision measurements, such as atomic clocks and gravimeters, and in quantum information processing, such as quantum cryptography and quantum computing.
Another important application of laser cooling is the trapping of particles in optical traps. Optical traps are created by focusing laser beams into tight spots, where the intensity of the light is very high. The particles are held in place by the gradient of the laser intensity, which exerts a force on them. Optical traps are widely used in atomic and molecular physics, as they allow particles to be confined and manipulated in a controlled way.
Key Laser Requirements
The laser system used in laser cooling experiments must meet several critical requirements.
Wavelength: One of the most important laser requirements is the wavelength of the light. The laser used for cooling must be resonant with the electronic transition of the atom being studied. This is typically accomplished by using lasers in the visible or near-infrared regions of the spectrum.
Power and Intensity: The laser power and intensity must be sufficient to counteract the thermal motion of the atoms and provide enough trapping force to keep them confined. This typically requires laser powers on the order of milliwatts to several watts, depending on the species being studied.
Spectral purity: The laser light must be monochromatic, meaning it should not have any sidebands or spectral lines that are not resonant with the atomic transition. Spectral purity is crucial because it ensures that the laser is only cooling the atoms in the desired state and not any others.
High Stability/Low Noise: Laser cooling experiments require highly stable laser systems. This stability is necessary to ensure that the laser is maintaining the same frequency and intensity over time, which is critical for maintaining the trap and cooling the atoms.
Beam quality: The laser beam quality is also important in laser cooling experiments. A high-quality laser beam, with a well-defined shape and size, is essential to ensure that the atoms are confined in a well-defined trap.
A number of different Coherent lasers types are useful in cooling and trapping applications, including SureLock diode laser modules. The most demanding laser cooling applications need the most stable lasers such as the Coherent Mephisto.
Read this whitepaper to learn more: