CUSTOMER SUCCESS STORY
University of Vienna: Shaping and Patterning Electron Beams with Laser Light
The Challenge
Dr. Thomas Juffmann is an Associate Professor at the University of Vienna (Austria) whose research group are focused on developing new imaging techniques in light and electron microscopy that “maximize the information extracted from each detected probe particle.” This research encompasses theoretical studies, multipass microscopy, adaptive optics, and optical near-field electron microscopy.
Dr. Juffmann explains that, in recent years, optical techniques in both microscopy and astronomy have benefited tremendously from the ability to manipulate photons using active components like spatial light modulators and adaptive optics. Electron microscopy is capable of providing uniquely high-resolution data about diverse samples, but it has not benefited from the same level of clever control over electrons.…yet. But a just published research study [1] by Juffmann’s group and collaborators at the University of Siegen has shown how that is now possible, with potentially huge implications for pulsed electron microscopy and metrology across numerous scientific disciplines. Potential examples cited by Juffmann include contrast enhancement in phase microscopy or ptychography with applications, for example, in observing phase transitions in solids.
The Solution
Juffmann and co-workers decided to focus on using the ponderomotive effect for this purpose, a weak scattering effect first predicted back in 1933 by Kapitza and Dirac [2]. The effect was finally observed by Bucksbaum et al. for the first time in 1988 thanks to their use of a pulsed laser [3], and later by Freimund et al. in a beautiful experiment that showed the diffraction of an electron pulse off a standing light wave [4]. Juffmann’s team set out to harness this fundamental mechanism to manipulate electron beams as never before.
How does it work? The pondermotive force refers to the movement of electrons in an oscillating electromagnetic field such as a beam of light that is not uniform in intensity. This force causes electrons to move away from high-intensity regions and into lower-intensity regions. Juffmann knew it could provide a way to manipulate electrons with light. However, this is also a weak effect that needs very high light intensity. So his group set out to create the necessarily intense field patterns using a femtosecond laser and a spatial light modulator.
The lab was equipped with a Monaco 1035 ultrafast laser which turned out to be an ideal light source for these experiments. Juffmann explains, “The combination of short (<300 fs) pulse width and high (40 µJ) pulse energy provides plenty of peak power for our current experiments, as well as future setups with more pixels in the electron patterns. And the 1 MHz pulse repetition rate translates into short data acquisition times.” He also cites the reliability of the laser as an advantage with no down-time in nearly 4 years of operation in his lab.
The Result
In the Juffmann setup, a beamsplitter picks off a few percent of the laser intensity. This is focused onto a metal tip to create a burst of electrons which are then accelerated as a collimated beam. The rest of the laser beam is patterned by a spatial light modular before it interacts with the electron beam in a counterpropagating arrangement. The figure illustrates the ability of this approach to create arbitrary electron beam shapes of virtually any geometry and detail: this shows an image of a phosphor screen irradiated by the electron beam that has been manipulated to create various patterns, including a “smiley face.”
Juffmann notes that in comparison to other electron manipulation techniques, this new method is programmable, and avoids losses, inelastic scattering, and potential instabilities due to the degradation of material diffraction elements. As a result, parts of your electron microscope in the future may include optical adjustments. Marius Mihaila, a Ph.D. student in the Juffmann lab summarizes, “Our shaping technique enables successful aberration correction and adaptive imaging in pulsed electron microscopes. It could be used to adjust your microscope to the specimens you study to maximize sensitivity.”
References
- MCC Mihaila et al, Transverse Electron-Beam Shaping with Light, Phys Rev. X 12, 031043 (2022). https://doi.org/10.1103/PhysRevX.12.031043
- P.L. Kapitza and P.A.M. Dirac, The reflection of electrons from standing light waves. Proc. Camb. Phil. Soc. 29, 297–300 (1933).
- P.H. Bucksbaum et al, High intensity Kapitza–Dirac effect. Phys. Rev. Lett. 61, 1182–1185 (1988).
- Freimund et. al, Observation of the Kapitza-Dirac effect, Nature, 413, 142-143 (2001).
“The combination of short (<300 fs) pulse width and high (40 µJ) pulse energy of Monaco 1035 ultrafast laser provides plenty of peak power for our current experiments, as well as future setups with more pixels in the electron patterns."
— Thomas Juffmann, Associate Professor, Department of Physics, University of Vienna, Austria
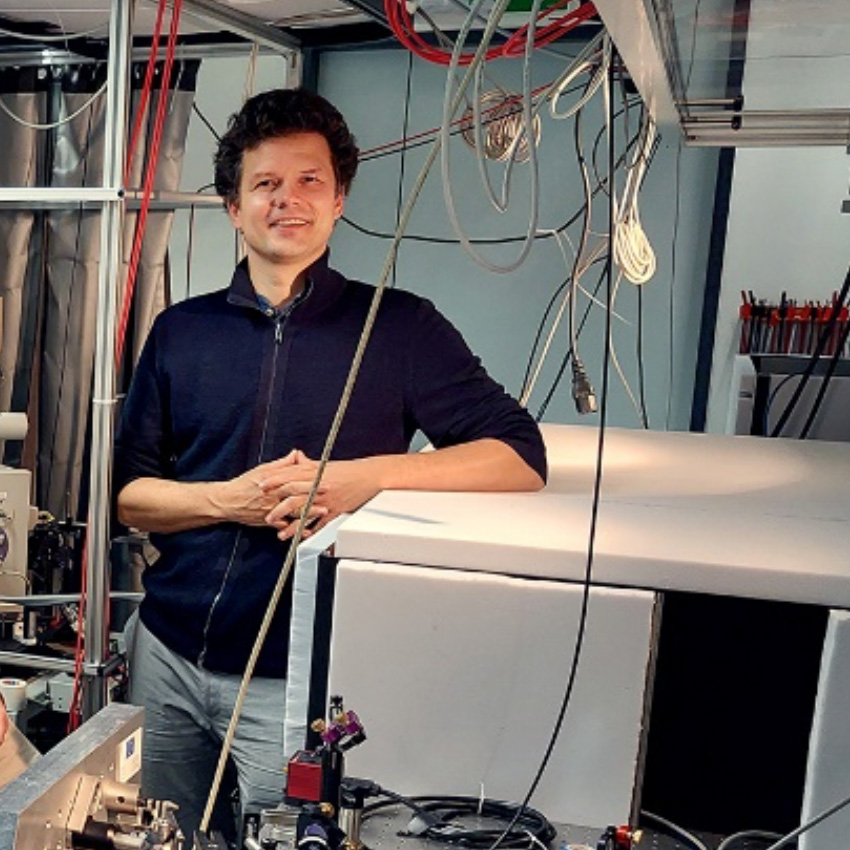
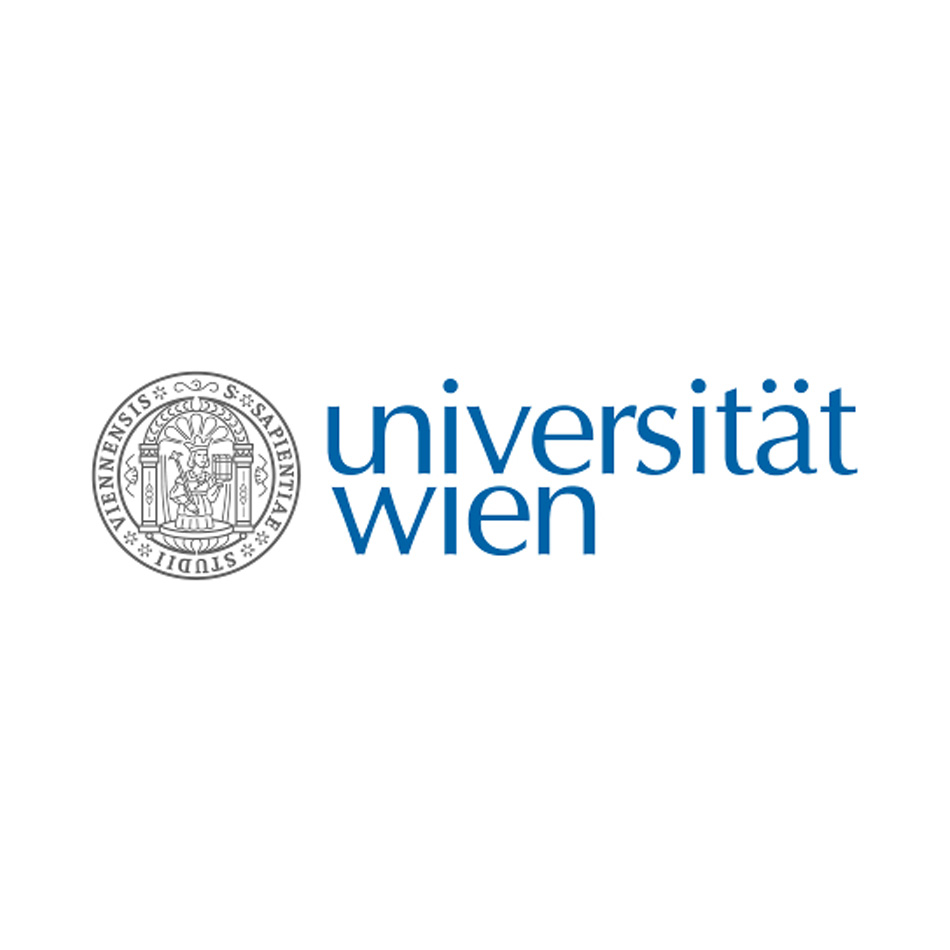
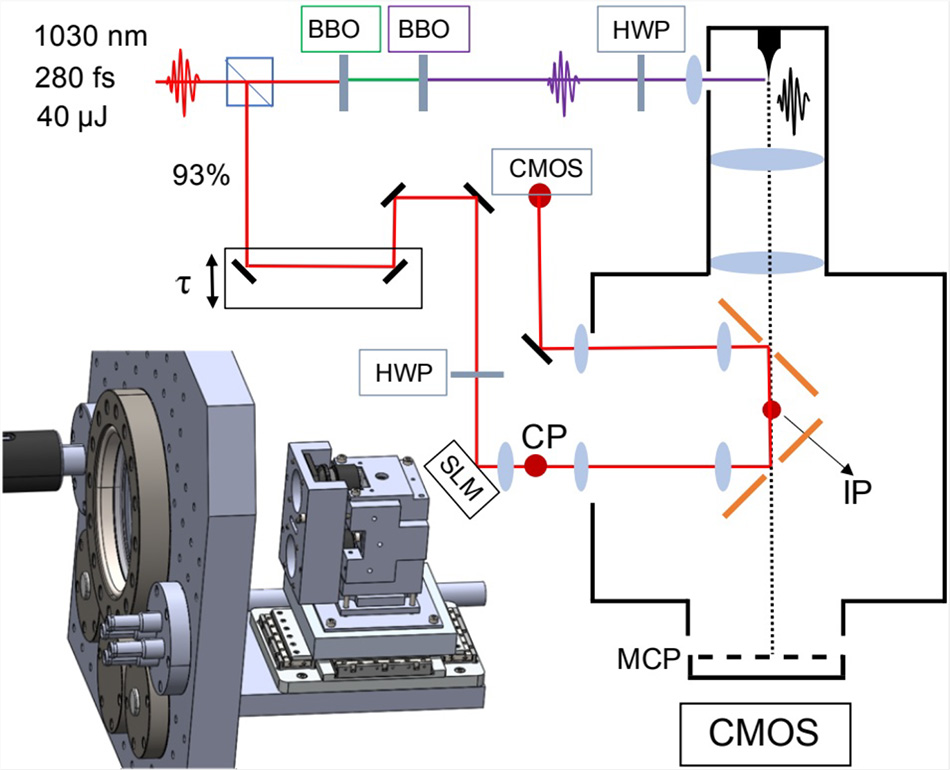
Figure 1. Schematic representation of the key elements of the electron beam shaping system. From [1]
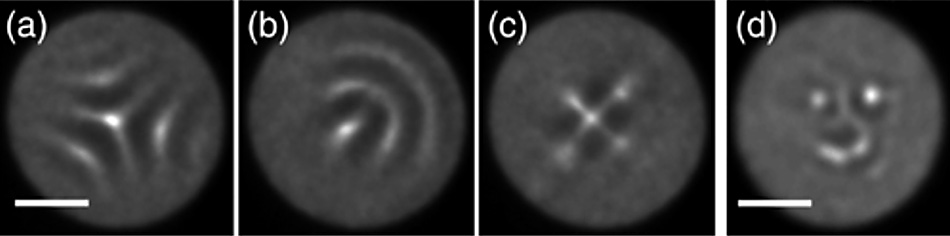
Figure 2. Images of a phosphor plate irradiated by shaped electron beams, including a smiley face. From [1].